Life has its shapes, and depends on all kinds of architecture. It needs a skeleton on which to hang. Blood vessels in which to flow. A brain to house its thoughts. A heart to give it a beat. On a far smaller scale, it needs cells to accommodate all the various components without which there would be no life in the first place. One of the most important being: DNA. DNA itself is found within a defined structure in each of our cells: the nucleus. Within this protective core, our genetic heritage adopts yet other variable conformations depending on a cell's stage in mitosis. One of these conformations is the well-known chromosome. Chromosomes are simply highly-packed DNA, which is an ideal conformation to be in when a cell is about to divide for instance, and chromosomes need to move around. Many different proteins work in unison to keep chromosomes arranged in such a way. One in particular has recently proved to be important in maintaining the shape of packed chromosomes, and is called proliferation marker protein Ki-67.
Why is it important for chromosomes to keep their shape in the first place? In the process of cell division, mammalian chromosomes evolve from an untidy slack conformation - bearing resemblance to boiled spaghetti - to the "X" conformation characteristically used to illustrate a chromosome. This "X" conformation is achieved when the DNA - or chromatin - of the slack chromosome is packed very tightly, making it far easier for the nucleus to identify chromosomes individually and distribute them correctly into the two daughter cells of a dividing cell. It is not dissimilar to folding clothing items thus making it easier to identify them one by one and tidy them away into drawers. Chromatin-packing may sound straightforward and perhaps even obvious, but it has taken well over a century to come to these conclusions and understand chromosome structure at the molecular level.
The German biologist Walther Flemming (1843-1905) was the first to observe what he termed "chromatin" because it was the substance in the cell nucleus that was "readily stained" under the microscope. At the time though, no one was yet aware of what its individual components were, i.e. DNA and protein. Nucleic acids had indeed been uncovered by the Swiss biologist Friedrich Miescher (1844-1895) in the 1870s already, but the notion of DNA as a "transforming principle" only emerged in the early 1940s and its structure was still to be unveiled. So, though the first half of the 20th century made huge leaps in the field of genetics, little was known on the molecular front.
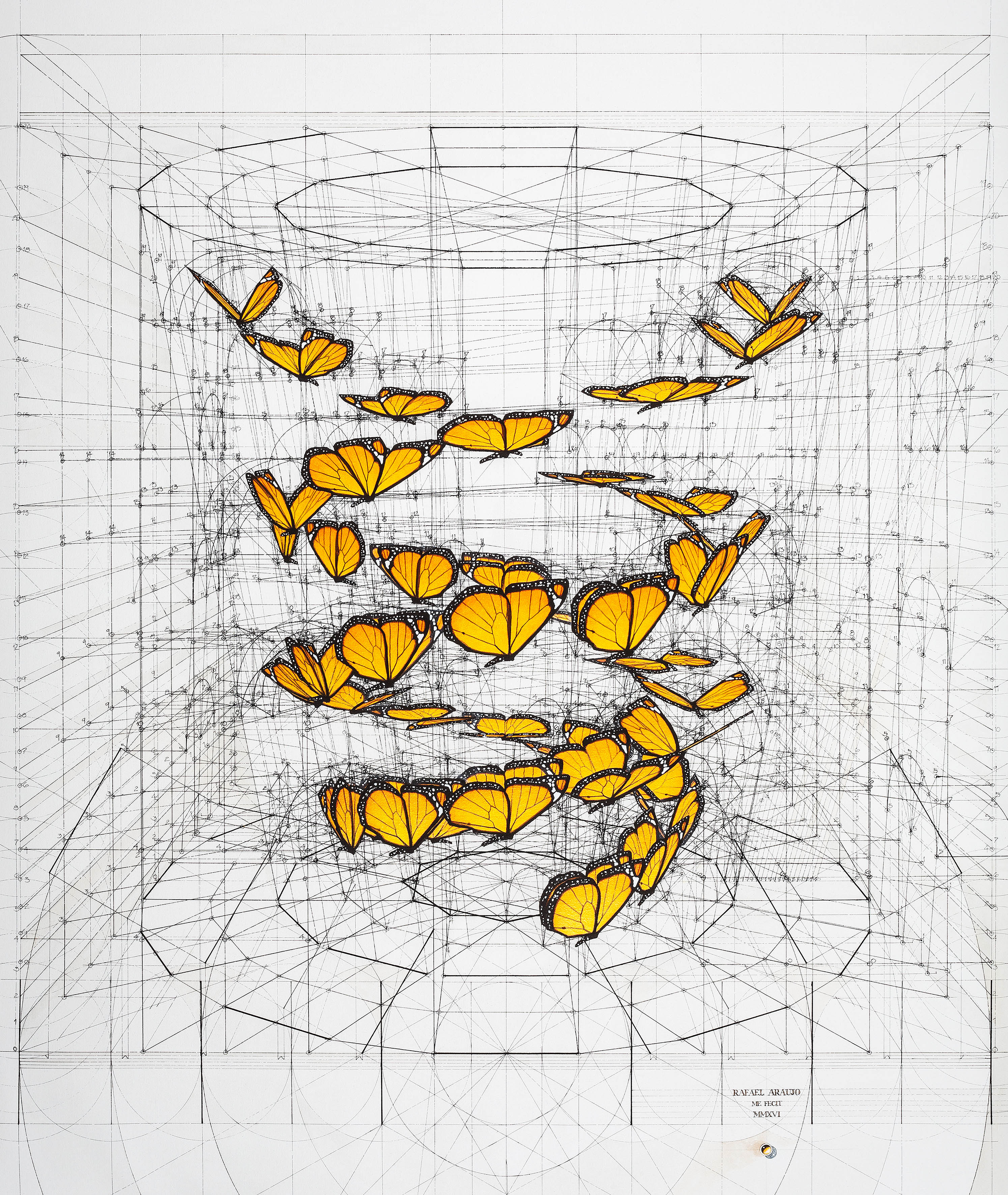
Courtesy of the artist
By the early 1940s, scientists knew that chromatin was a mixture of DNA and histone proteins. Intriguingly though, since DNA seemed to be so monotonous from a structural point of view, the consensus was that the histones must be the carriers of genetic information. This notion soon shifted, however, when the double helix structure of DNA was reported by the American biologist James Watson and the English physicist Francis Crick (1916-2004) in 1953. Finally, during the 1970s and the 1980s, the discovery and the role of what has been called the "quantum of chromatin" was described: the nucleosome. Made of a histone core around which is coiled a given stretch of DNA, nucleosomes form the structural unit of chromatin packaging. They are responsible for its tight packing into the shape of chromosomes, and are therefore of great significance in gene expression.
Proliferation marker protein Ki-67, or Ki-67, does not help to pack DNA into chromosomes, but it does prevent them from losing their shape. How? KI-67 is a nuclear-binding protein in mammalian cells. It is very large, has a very high electrical charge and seems to exist in a long unfolded conformation. Its structure is amphiphilic, meaning that one side is negatively charged, while the other is positively charged - typical features of surface-active agents. Ki-67 is hugely expressed during cell division - the time when the nucleolus disassembles to release its chromosomes and share them equally between two nascent daughter cells. It is precisely at this point that Ki-67 becomes essential by forming a sort of sheath around each mitotic chromosome making sure that everything stays tightly packed and neatly in place.
A closer look at this sheath reveals a dense fuzzy brush-like structure that runs around the contour of each chromosome. On closer inspection, each "hair", so to speak, is formed by a Ki-67 protein, whose C-terminus is attracted to chromatin while its N-terminus prefers the cytoplasm. This creates a sort of ephemeral electrostatic "exoskeleton", a little like a suitcase into which clothes have been stashed for a journey. Once over, the suitcase is opened, and the clothes released. This is precisely what happens to the Ki-67 sheath. When cell division has occurred, and the chromosomes have been distributed between the two nascent cells, the nucleolus reassembles to contain them. The Ki-67 sheath then dissolves, and the chromosomes adopt their untidy slack spaghetti-like conformation as they await the next cell cycle.
So Ki-67 holds chromatin in a highly compacted form while, no doubt, mediating long-range interactions between different parts of the chromosome. Furthermore, since each outside shell has the same electric charge, instead of getting hopelessly tangled, chromosomes bounce off each other! This is a delightful example of the biomechanical role of a protein. This said, though essential, Ki-67 does not work on its own: at least seventeen other proteins are known to be involved in the process too. It could be that Ki-67 targets these proteins to their correct sites in the process of compaction. Time will tell. With all this in mind, it is not surprising that Ki-67 is used as a biomarker in the prognosis of cancer. Cell division is highly controlled, and only certain cells should be dividing at a given time in our body. Ki-67 can be used to tell if the wrong cells have indeed lost control, as can other biomarkers within the nucleolus. In this light, inhibitors of nucleolar functions have been shown to destroy cancer cells selectively, and getting to know them in greater detail will help in the never-ending fight against an affliction that kills far too often.